Jeremy Brown
Professor, Department of Electrical and Computer Engineering, School of Biomedical Engineering
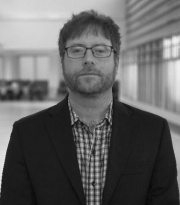
Our lab designs and fabricates high-frequency array-based ultrasound transducers and electronics, with applications ranging from small animal imaging to neurosurgical guidance. We operate a clean room fabrication facility at the Nova Scotia Health Authority where ultrasound probes are designed, built and tested. We also design an fabricate a wide variety of low frequency ultrasound transducers for therapeutic applications.
Our Group
Research in our laboratory is focused on the design, fabrication, and testing of ultrasonic frequency piezoelectric transducers and associated electronic hardware for medical applications. Specifically, we are focused on developing miniaturized ultrasound imaging endoscopes and miniaturized ultrasound therapeutic devices using an in house micro-fabrication facility targeting minimally invasive surgical applications.
Minimally invasive surgical approaches in comparison to open surgery, offer drastically improved patient outcomes including less blood loss, fewer complications, reduced recovery time, and a reduced chance of infection. To allow more procedures to be performed as minimally invasive, it’s important to develop new surgical tools specific to the minimally invasive surgical procedure. As these procedures are almost always performed through a very small incision or access route, the associated tools must be developed in a miniaturized form factor.
Projects
Neuroimaging endoscope Typical high-frequency ultrasound resolution of 30-100 microns can be achieved in soft tissue over a penetration depth of 10-20 mm. The short penetration depth and high resolution, make high-frequency ultrasound particularly suitable for use in guided endoscopic surgery. In these minimally invasive procedures, a set of surgical instruments are inserted into a small incision site along with a set of imaging tools, typically endoscopic optical cameras and light sources. The entire surgical procedure is done solely under image guidance. Such an approach has become standard of care for a very large number of surgical procedures including those of the brain, colon, pancreas, uterus, bowel, etc. Our group has developed a high-frequency array-based forward-looking ultrasound endoscope, that is suitable for guiding endoscopic procedures and providing depth resolved information. The packaged form factor for this imaging array has been miniaturized down to just a few millimetres. We are currently evaluating the miniature high resolution imaging endoscope in a series of pre-clinical in-vivo neuroimaging studies. |
|
Miniature Histotripsy Transducer Currently, two ultrasound based non-invasive surgical techniques are available: high-intensity focused ultrasound (HIFU) and histotripsy. Where HIFU uses a highly focused ultrasound transducer to cause tissue necrosis through thermal means, histotripsy, using a similar transducer, causes tissue fractionation through short bursts of high pressure pulses, where the destruction is caused by inducing and collapsing cavitation bubbles. Histotripsy, being a relatively new area of ablation research, has just started the first rounds of human clinical trials, however, this form of non-invasive ultrasonic surgery has been repeatably demonstrated in pre-clinical studies. Virtually all histotripsy has been performed with very large (>10 cm) low frequency ultrasound transducers in contrast to the miniature (<5 mm) histotripsy endoscopes that we are developing. One of the most common methods of tumor therapy in the brain is through minimally invasive surgery in which a small ~20 mm hole is made in the skull and, using optical guidance as well as pre-operative MRI images, a miniature surgical ablation device is used to erode the tumor tissue. Although effective, the surgeon cannot simultaneously collect depth resolved images and ablate tissue at the same time, and since the ablation happens upon contact with the tool, sensitive tissues and vessels can not be spared if they lie in the path of the tumor access route as they are with our histotripsy device. |
|
2D Arrays for 3D Ultrasound Imaging 3D Ultrasound systems based on 2D matrix arrays present several technical challenges, particularly the large number of elements in the 2D array, high electrical impedance, and image acquisition time. An alternative approach to 2D matrix arrays are a new type of array consisting of a set of linear array electrodes on one side of a piezo electric, and an orthogonal set of crossed electrodes on the opposite side of the piezoelectric. Crossed electrode arrays address some matrix array issues, especially the huge reduction in number of elements. However, creating a two-way focused 3D image in real-time is difficult with these arrays because azimuth and elevation dimensions cannot be beamformed at the same time. This typically forces one to use a synthetic aperture approach which is inherently slow and requires increased beamforming complexity over a 1D array. We have developed a new, fast and simple 3D imaging approach for a new type of crossed electrode array. The high level principle behind this technique is to perform compound imaging using the top set of electrodes, while at the same time compounding a reconfigurable Fresnel elevation lens with the orthogonal bottom electrodes. This allows one to produce a highly focused beam in both orthogonal dimensions simultaneously. We have demonstrated that this form of simultaneous compounding can produce high quality 3D images at real-time frame rates. |
Selected Publications
C.A. Samson, A. Bezanson, J.A. Brown, “A Sub Nyquist, Variable Sampling, High Frequnecy Phased Array Beamformer,” IEEE Transactions on Ultrasonics Ferroelectrics & Frequency Control, (In Press, manuscript no. TUFFC-07930-2016, published online with IEEE early access Dec. 2016). |
T. Landry, J. Rainsbury, R. Adamson, M. Bance, J.A. Brown, “Real-Time Imaging of the Human Middle Ear,” Hearing Research, Vol. 326, pp. 1-7, 2015. |
J.A. Brown, S. Sundaresh, J. Leadbetter, A. Bezanson, S. Cochran, R. Adamson, “Mass-Spring Matching Layers for High Frequency Ultrasound Transducers: A New Technique Using Vacuum Deposition,” IEEE Transactions on Ultrasonics Ferroelectrics & Frequency Control, Vol.. 61, pp. 1911-1921, 2014. |
Y. Zhang, P.Garland, R. Adamson, J.A. Brown, “The Quenched State with Dominant Shear Vibration Mode Originated from Domain Reconfiguration in [001] Oriented PMN-PT Single Crystals,”Journal of Applied Physics, Vol. 115, no. 21, pp. 214101-214101-10, 2014 (Featured Cover Article). |
A. Bezanson, R. Adamson, J.A. Brown, “Fabrication and Performance of a High Frequency Forward Looking Phased Array Endoscope,”IEEE Transactions on Ultrasonics Ferroelectrics & Frequency Control, Vol. 61, pp. 33-43, 2014. |
Z. Torbatian,R. Adamson, J.A. Brown, “Experimental Verification of Pulse probing Technique for Phase Coherence Grating Lobe Suppression,”IEEE Transactions on Ultrasonics Ferroelectrics & Frequency Control, Vol. 60,pp. 1324-1332, 2013 (Front cover article). |
A. Bezanson, A. Adamson, J.A. Brown, “Fabrication and Performance of a Miniaturized 64 Element High Frequency Phased Array,”Proc. IEEE Ultrasonics Symposium,pp. 765-768, 2013. |
Z. Torbatian, P.Garland, R. Adamson, M. Bance, J.A. Brown, “Listening to the Cochlea with High Frequency Ultrasound,”Ultrasound in Medicine and Biology, Vol 38:12, pp. 2208-2217, 2012. |
R.W. Deas, R. Adamson, L.L. Curran, F.M. Makki, M.L. Bance, J.A. Brown, “Audiometric thresholds measured with single and dual BAHA motors: the effect of phase,” International Journal of Audiology, Vol. 49(12), pp. 993-999, 2010. |